Project Pete

High Voltage DC Energy Storage
I once went to see a talk about renewable energy where someone in the audience asked a really interesting question which went along the lines of:
​
'why do we bother with energy storage when we could just put massive cables around the planet? Which ever side is sunny will be generating enough electricity for the dark side with the roles reversing every 12 hours or so!'
​
It's an appealing vision though not without major technical, environmental and geopolitical challenges. That being said, High Voltage DC (HVDC) transmission technology may one day make this vision a reality. But why use DC to transmit power when the war of the currents was so decisively won by AC back in the late 19th century? Over very large distances, HVDC starts to become appealing for a number of reasons including:
​
1. You don't need as many cables compared with AC
​
2. You carry more energy with a lower peak voltage which dictates your insulation requirements (an AC line may have an RMS voltage of 270kV, it's peak is up at 380kV)
​
3. In AC lines you get losses due to the skin effect which is caused by the tendency of alternating currents to migrate to the edge of a conductor, effectively reducing the available conductor volume for power tranmission.
​
Another issue is that over very long distances, the parasitic capacitance between the conductors in an AC line start to become a significant loss as it is charged and discharged. My good friend Khalid and I decided to explore an idea around whether this parasitic capacitance could actually become a meaningful source of energy storage. In a HVDC line, parasitic capacitance doesn't cause any real harm, however by moving the operating voltage of the line up and down, could this capacitance become a meaningful source of energy storage? If it could you'd have the benefit of using one piece of infrastructure for two purposes, bulk power transmission and energy storage. Moreover you might not have to move the voltage around that much, given that the energy stored goes with the voltage across a capacitor squared and we're talking pretty huge voltages here!

Figure 1 System diagram of scheme
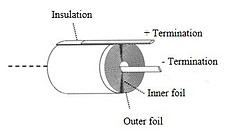

Figure 2 Cross sections of proposed cable

Figure 3 Trying to design cable in a way that avoids arcing
The system layout is captured by Figure 1 which is a basic HVDC line with a huge capacitor stuck across it. When we looked at the spec of conventional HVDC cables we quickly found there's nowhere near enough capacitance to deliver any meaningful amount of energy storage. So we set about designing a cable with the express purpose of delivering massive amounts of capacitance. The cable would be a pair of conductors arranged in a spiral wound foil with a dielectric sandwiched between them (see Figure 2). The big challenge was around finding a dielectric which had: 1) a really high permittivity, to store lots of energy, 2) good electrical breakdown strength to stop electricity arcing across the foils and 3) very high resistivity so that energy losses wouldn't be too great. It turned out that there weren't any practical materials to hand at the time. The closest readily available materials would probably involve composite Mylar sheets (having lots of thin sheets is better for high electrical breakdown strength than one homogenous material). Even so, the cable would end up being impractically big. There were however some very interesting papers given to us by Professor Pete Dobson (former head of Oxford University's Begbroke Science Park) which described lab materials with enormous permittivity values. In particular, a class of Barium Titanate glass composites exhibited the combination of 3 properties above.
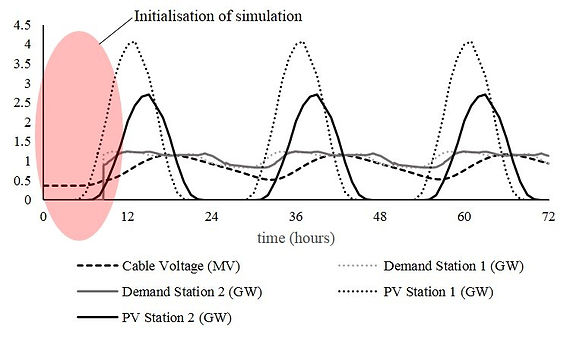
Figure 4 Simulation of our highly impractical Morocco to Egypt HVDC line
We decided therefore to design a hypothetical cable which could store 10GWh of energy along a 1MV HVDC line from one side of North Africa (Morocco on the West) to the other (Egypt in the East). We assumed there'd be a large solar PV farm at each connection point and wanted enough energy storage to allow all of the local demand at each station to be satisfied by the solar energy 24 hours a day. Interestingly you get a little help from the temporal shift in solar generation profiles (along the lines suggested in the very opening paragraph), however you still need a colossal amount of energy storage! By this point the parameters were just getting a bit silly as we were now talking about a cable that was about 1.5m in diameter using vast amounts of expensive dielectric materials. The biggest and most intractable problem was around the danger posed by such a massive capacitor. If any section of the cable's dielectric were to be compromised, you'd have a sudden discharge of energy which would be like the mother of all lightning bolts. This would surely blow a huge hole in the cable which would mean you'd have exposed coils arcing in fresh air (the design required the foils to be separated by only 30mm to achieve enough capacitance, this is no where near enough to stop 1MV from tracking the surface of exposed dielectric resulting from the initial explosion. I think we worked out the amount of energy in this exploding cable would be a equivalent to about 1 stick of dynamite per meter. We could see no viable way of stopping the entire cable from exploding under these circumstances without the scheme becoming uneconomic (if it wasn't already).
Concluding thoughts
Looking back, I think we were probably being a bit too ambitious in trying to store such a colossal amount of energy for balancing a 100% renewable generation scenario. It may be more practical to add smaller quantities of capacitance for short term energy storage services such as frequency response. Adding 1GWh to the UK-France 2GW HVDC might one day be feasible and could deliver almost all of the UK's frequency response requirements in theory, somehow I doubt National Grid would want to put all of their eggs into such a dangerous basket, atleast if anything went wrong the explosions would be for the most part undersea.