Project Pete
Thoughts on thermal stratification
The way thermal energy behaves in water is quite remarkable. It's often pointed out that ice is a very unusual substance because it expands as it solidifies, but another interesting property of water is the way in which it can float when heated. This topic became a big part of my PhD work on energy storage systems as I became interested in the physics of stratified heat within domestic hot water tanks and the consequence for storing renewable power (see a link to my thesis here if you want to explore that rabbit warren further!).
As I learned more about thermal stratification I came across a number of other areas where the subject cropped up. One interesting example relates to the ecology of deep lakes where aquatic life is heavily influenced by the vertical temperature gradient throughout the year. The temperature influences the amount of dissolved oxygen which in turn affects the presence of plankton and associated nutrients. Over the summer, a strong thermocline emerges between the hot surface and cold depths, this turns on it's head quite suddenly as temperatures drop in winter mixing all of the water in the lake. In Dimicitic lakes (common in temperate climates), these mixing events occur twice a year and can result in algae blooms as nutrient rich sediment is brought up to the surface. Some lakes stay in a permanently stratified state (Amicitic) or permanently mixed state (Holomicitic)
​
My interest in all of this focused on how to predict the evolution of a temperature gradient in a fluid and the sorts of behaviour that might occur. Stratified heat within water can stay concentrated and undisturbed for a long time due to water's low conductivity and the strong buoyancy forces that result from thermal expansion as the temperature rises above 4 degrees Celsius. As water chills beneath 4 degrees you have thermal stratification in reverse, with chilled water floating on top of warmer layers. The following diagram shows how both of these processes occur in Dimicitic lakes which capture the full range of water's curious behaviour (well in a liquid state at least!):
​
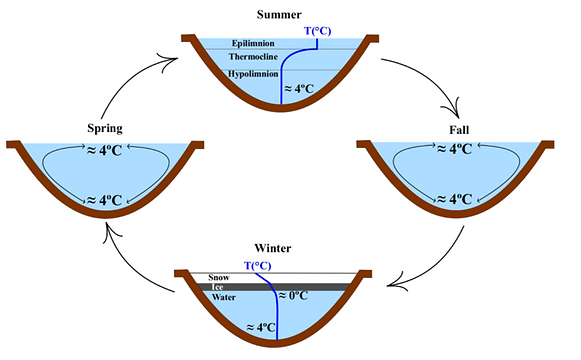
This image is from a report by Celeste Irambona et al about Lake Ontario but is originally derived from Shaw B, Mechenich, Understanding Lake Data (2004)
Modelling Thermal Stratification
In order to get a handle on how thermal stratification works, I got into the subject of Computational Fluid Dynamics (CFD). Essentially CFD is all about breaking a geometry down into small elements and applying a set of equations to each element called the Navier Stokes Equations. The following diagram shows three different ways of doing this (either with a finite element moving along or a control volume with flux moving through it).
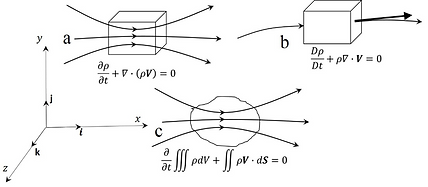
Different ways of formulating the governing equations in CFD in Cartesian coordinates

Numerical results showing velocity vector field and temperature scalar field within a hot water tank radial cross section. Note the counter rotating vortices above and below the thermocline
To apply CFD to a particular geometry, you have to break it into thousands of tiny cells (a process called meshing). This is a very deep subject in and of itself as there are all sorts of trade-offs around mesh density versus computational speed, numerical errors not to mention the overlap between the bulk fluid and enclosing surfaces via interpolating wall functions. In many ways it becomes a bit of a 'dark art' though I'm sure more qualified CFD experts would be horrified to hear me say this!
​
I was particularly interested in how a thermocline interacts with a conductive surface and whether this would undermine water's ability to retain stratified heat in a domestic hot water tank. The longer the tank could hold heat at a useful temperature for, the more useful it would be at bridging the periods where renewable energy is relatively available or not. The images to the right show the numerical results which showed that the heat conducted down the tank wall set-up a pair of counter rotating toroids (see more detail in a paper published in Applied Energy here ).

Schematic explanation of the rotating vortice toriod system established in a tank
This was pretty cool to get to see a new phenomenon that couldn't be easily captured in an experiment but comes alive during simulation. Whilst there are very good experimental visualisation techniques (such as particle image velocimetry), applying these to a 3d flow structure would be very difficult (in fact please get in touch if you have a way to experimentally visualise this!). However, it was possible to experimentally verify the macro effect of this phenomenon by building a test tank and taking temperature measurements around a thermocline that was established by a heating element positioned half way up the cylinder. The two images below show the detail of this tank on the left and me at the factory the test tank was made on the right (holding a prototype diffuser used in another experiment discussed below.
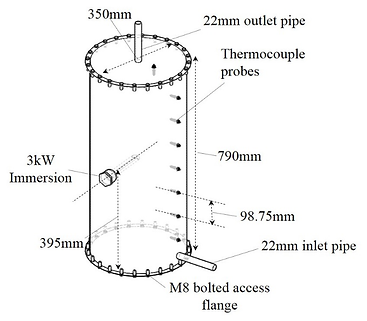

Test tank (left) built to validate numerical work above and me (right) holding a prototype diffuser at Newark Copper Cylinders
The test tank was made at Newark Copper Cylinders in Nottinghamshire. I'll always be indebted to the owner of the factory, Mark Smith (who happens to be a world expert on Japanese motorcycles), who provided all sorts of help for free during my PhD. Newark also made our first production cylinders at Mixergy and remain a great source of help for prototyping and getting industry advice.
The diffuser I'm holding in the picture above was used to stop mixing (or de-stratification) within a hot water tank during times when cold water is rushing in to the bottom as the system is being used. This was another area where CFD was able to shed some insights, for instance the following images show the evolution of a cold plume as it flowed into a fully heated test tank.
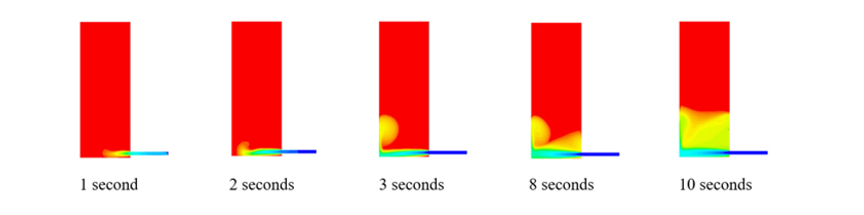
Evolution of a plume in a 300mm diameter cylinder at 15lpm through a 22mm inlet pipe
Simulating the plume above was interesting since it involved a combination of both thermal stratification along with turbulence. Turbulence is ubiquitous across engineering applications and occurs wherever the Reynolds number exceeds a figure of 3,000 – 4,000. To capture the effect of turbulence, we need to introduce “pseudo-stress” terms in the governing equations. These stresses arise due to the viscous friction between a chaotic assortment of eddies and vortices that occur down to very small length scales, eloquently described by the following poem:
'Big whorls have little whorls
Which feed on their velocity
And little whorls have lesser whorls
And so on to viscosity'
​
Lewis F Richardson
Capturing turbulent shear stresses within a generalizable model is one of the last challenges of classical physics with a £1million Clay Institute prize for whoever is successful. For now we make do with turbulence models (such as the k-ε model) which approximate their behaviour but still always need to be experimentally validated.
Concluding Thoughts
Thermal stratification continues to be interesting at Mixergy although CFD is a very expensive tool to use to understand it (both in terms of cost and time). So we continue to make use of a lot of experiments in addition to simpler analytical and numerical models (usually via a 1 dimensional approximation). Whilst I really like the visual insights that CFD brings, I feel that analytical models should always be attempted first as these are more likely to allow you to truly determine what's important before generating lots of data. I think things work best when you have a good analytical model which is calibrated by CFD simulations that are validated by experiment! This is the stuff of good journal papers and is rare to find problems where you can achieve all those things but good fun if you can.
​
It would be very cool to hear from anyone who can set-up an experiment for us to see these elusive counter rotating thermocline toroids or equally from anyone who is an expert in the thermal behaviour of lakes and the consequences on their ecology, it would be interesting to know more about how they research thermal stratification and whether they have any better/faster models!